The task of science is to stake out the limits of the knowable, and to center consciousness within them.
Rudolf Virchow
The Continuing Education article by Spagnoli et al. on pages 1800–1815 of this issue of The Journal of Nuclear Medicine (1) provides an excellent review of the pathophysiology of atherosclerosis, focusing on the major role of inflammation in the genesis, progression, and symptomatology of the disease. The American Heart Association (2) indicates that the term atherosclerosis comes from 2 Greek words: athero, meaning gruel or paste, and sclerosis, meaning hardening. Although the process was recognized See page 1800
centuries ago (3), Rudolf Virchow, the 19th-century German pathologist, suggested that inflammation played an important role. An alternative view, espoused by the Austrian pathologist Carl von Rokitansky, suggested a role for blood (especially fibrin) in the pathology of atherosclerosis (4,5). It is now apparent that both of these renowned pathologists were correct: A combination of the “vulnerable plaque” and the “vulnerable patient” contributes to a clinical event. A recent analysis of tissue specimens from autopsies performed by von Rokitansky nearly 2 centuries ago demonstrated CD3-positive cells in early lesions, suggesting that the role of inflammation in the pathophysiology of atheroma has not changed since early descriptions of the disease. As Spagnoli et al. (1) indicate, inflammation plays a major role in atherosclerosis, especially in the coronary arteries. They also point out that atherosclerosis is a diffuse disease, with multiple plaques capable of causing clinical events, although often only one culprit lesion causes a clinical event. Plaques go through multiple cycles of lipid infiltration, inflammation, progression, and healing. These cycles are associated with the formation of small, clinically silent, nonocclusive thrombi, endogenous thrombolysis, resorption, reendothelialization and, at times, calcification (6,7). This cyclic process is associated with numerous signaling pathways (e.g., integrins, chemokines, angiogenesis, and apoptosis), cell types (e.g., monocytes, macrophages, mast cells, and lymphocytes), and metabolic events. Each event offers an opportunity for detection and characterization with radionuclide imaging.
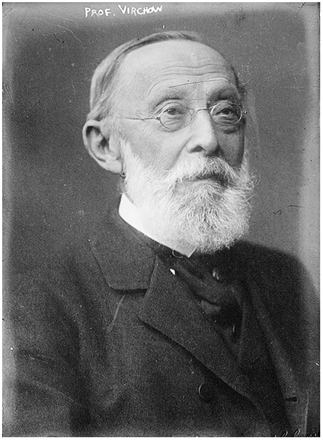
Virchow

Rokitansky
The following is an incomplete list of agents that have been reported to concentrate in experimental or human atheromas and their mechanisms of localization. There are 4 broad categories of radiolabeled agents:
Lipoproteins (8,9), methylated (10) and oxidized (11) lipoproteins, and antibodies recognizing oxidized low-density lipoprotein (12,13);
Markers of inflammation: upregulated integrin expression (14), endothelin receptors (15–17), IgG (18), chemoattractant peptide expression (19,20), macrophage metabolism (18F-FDG) (21–25), macrophage function (e.g., phagocytosis (26) and markers of matrix metalloproteinase expression (27,28)), and extradomain B of fibronectin (29);
Markers of cell death (e.g., especially of macrophages and smooth muscle cells, which can be identified with annexin V (30–32));
Antibodies recognizing a change in the smooth muscle phenotype (33,34) (the smooth muscle cells found in atheromas are different from normal vascular smooth muscle cells; in normal tissue, the smooth muscle has a contractile phenotype, whereas in plaque, the smooth muscle has a proliferative phenotype; this phenotypic change is accompanied by a change in the markers on the cell surface, allowing identification of these cells with an antibody recognizing this epitope).
The complex, repetitive cycles of atherosclerosis make it difficult to select the most appropriate marker for identifying patients at risk for imminent events. Identifying lipoprotein and oxidized low-density lipoprotein in an atheroma may be useful in identifying the site of an atheroma but is not likely to offer a differential signal between stable and vulnerable lesions. On the other hand, markers of moderate to severe inflammation, such as macrophage metabolism, integrin expression, or chemotactic receptor expression, are more likely to distinguish stable from unstable lesions. There has been substantial clinical interest in 18F-FDG imaging of atheromas because it is widely available and there is an infrastructure of evidence about 18F-FDG uptake and inflammation (35–37). These facts alone make a compelling argument to investigate 18F-FDG as a marker of vulnerable plaque. There would be no waiting for the approval of a “new” drug. In addition, there are preliminary studies demonstrating the reproducibility of carotid 18F-FDG uptake over a 2-wk interval (38) and studies in animals and humans demonstrating a reduction in vascular 18F-FDG uptake as a result of drug therapy (39,40). Even with these exciting data, it is clear that vascular 18F-FDG imaging needs further development before it achieves its clinical potential. The most important parameters will be patient preparation, standardization of the time of imaging, gated image acquisition, and quantitation of vascular 18F-FDG uptake.
To detect 18F-FDG uptake in the coronary arteries, there must be essentially no myocardial 18F-FDG uptake. Elimination of the uptake typically seen in the myocardium requires either a pharmacologic or a dietary intervention (41,42). Although prolonged fasting has been suggested, there is no definite evidence that this intervention reliably suppresses myocardial uptake (43–45). Further clinical testing is required to develop a method that will eliminate myocardial uptake in all patients.
Although maximum vascular lesion contrast in the carotid arteries appears to occur at 3 h (46), the majority of uptake usually occurs in the first 60–120 min. Assuming that lesion uptake is fixed by 2 h after injection, the lesion count rate is reduced by about one third by waiting an additional hour for enhanced contrast before recording the data. Although activity in the blood pool is reduced, there is a marked degradation in image quality at lower count rates. These data make a relatively compelling argument to image patients at about 2 h after injection.
Cardiac gating is critical for minimizing the motion of the coronary tree. As with coronary CT angiography, the lower the heart rate, the better the result, because the coronary arteries move least during diastole. At a heart rate of 60, a 16-frame gated acquisition should be adequate. The data should be viewed both as a gated acquisition and with extraction of the approximately 4 usable frames at middle to late diastole that have minimal blurring and that can be summed to enhance the count density of the coronary arteries.
With regard to quantitation, at least 4 factors will have to be considered: partial-volume correction with contemporaneous CT, the intensity of uptake in each lesion (maximum standardized uptake value after partial-volume correction), the number of sites in a specific vascular territory that have uptake over a threshold, and the total number of vascular territories with 18F-FDG uptake in the coronary arteries and cerebrovascular bed.
The intensity of uptake correlates with the number of macrophages in a lesion (37), which should be a major indicator of lesion severity. The number of sites that have uptake over a threshold is important because there are usually multiple vulnerable plaques (47,48) in each vascular territory. Summing the total number of lesions should provide evidence for risk in a specific patient.
Integrating additional indicators, such as clinical risk, with the imaging score should add to the predictive power of the study. Similarly, integrating information about a vulnerable patient, such as the likelihood of thrombus formation on a specific lesion (49), should add another dimension to the measurement.
Other 18F-labeled tracers, such as 18F-labeled Ap4A (50), which may identify other characteristics of a vulnerable lesion, are under investigation. It is clear that molecular and anatomic imaging of vulnerable plaque will use a multimodality and multitracer approach. In the next 10 y, it is likely that a comprehensive characterization of the risk of a cardiac event will be developed. This characterization will include the results of vulnerable plaque scoring, serum lipid and inflammatory markers, global and regional perfusion reserve, myocardial ischemia or scar, the presence of endothelial apoptosis (the likely cause of plaque erosion), and the balance of cholinergic and adrenergic receptor expression (as a marker of possible arrhythmia).
An enhanced understanding of the major role of inflammation and the array of radionuclide imaging procedures for localizing the site(s) of these processes suggest that imaging of vascular inflammation will be an important component of patient care in the next decade.
Footnotes
-
COPYRIGHT © 2007 by the Society of Nuclear Medicine, Inc.
References
- Received for publication August 8, 2007.
- Accepted for publication August 17, 2007.